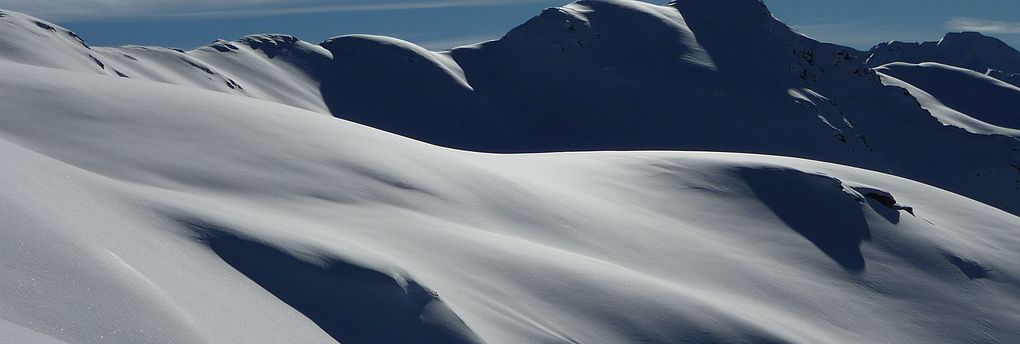
Snowpack
Without snowpack there would be no avalanches. But snowpack also provides a surface for ski runs, protects flora and fauna and induces a cooling effect through snow-atmosphere coupling. These are just a few examples of its significance but they show how important snowpack is to both humans and the natural world.
Snowpack consists of layers, rather like a cream slice. Each snowfall adds another layer. Snowpack therefore tells the history of a winter, from the oldest layers on the ground to the most recent on the surface. Some layers are so distinctive that weeks later we can link them back to specific weather events, e.g. a thick melt-freeze crust to a warm snap. Weak layers, such as snow-covered surface hoar, can increase the risk of avalanches. SLF observers therefore monitor their development closely. To improve avalanche risk forecasting, we are working to better understand and reproduce snowpack structure and the processes that occur inside it.
Changeable snowpack
Snowpack is a master of transformation: a person might sink up to their waist or walk over the top, depending on its condition. This is the result of continuous changes in the snowpack itself, most of which cannot be seen from outside. The temperature has a particularly big influence on these changes. The warmer the snow and the greater the temperature differences between the snow surface and the ground, the faster the snow layers change.
The wind also shapes the snow surface (Fig. 1). It transports the snow grains and grinds them down. It forms hard wind slabs. It blows snow from summits and ridges and fills up hollows and channels, rendering traffic routes impassable.
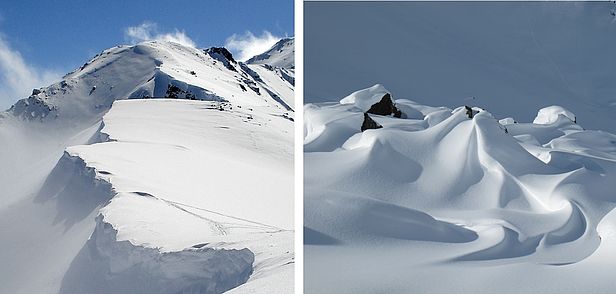
We investigate the fundamentals of snow drift in detail at the SLF's wind tunnel facility and at test sites. The measurement results feed into computer models, which simulate snowpack properties.
Radiation
The specific physical properties of snow are also important in snowpack. The dazzling white of a fresh snow surface clearly shows that snow has a high diffuse reflectivity (albedo). In the visible range, 80‑90% of incident solar radiation is reflected back into the atmosphere, compared with 20-50% for a coniferous forest and just 5-25% for a calm water surface. This means that snow only absorbs a small fraction of solar energy compared to other surfaces. The little energy that does penetrate it affects the snow’s metamorphism.